February 15, 2022
Hyuk Kim
The following was originally published on 38 North.
North Korea’s nuclear ambitions have served two purposes: nuclear energy for self-sufficiency and nuclear weapons for military power. This has been demonstrated through its current fission-based nuclear activities—producing fissile material and conducting six underground nuclear explosion tests. Since at least 1991, however, there has been evidence in North Korean media and scientific journals, such as the Journal of Kim Il Sung University (hereafter “the Journal”), that the country has been pursuing nuclear fusion as well. This is another dual-use technology that could be used for both civilian energy production and acquiring weapons-related capabilities.
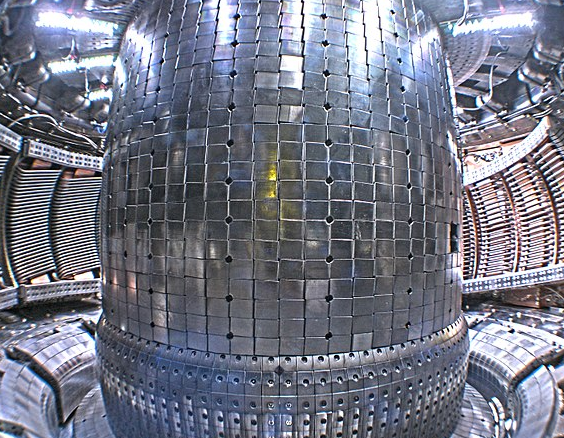
Tokamak Interior, Source: Mike Garrett via WikiMedia Commons
Of its many possible applications in the area of fusion research, inertial confinement fusion (ICF) helps states with experience in nuclear testing advance their weapons program without having to conduct further full-scale testing. North Korea began working on an ICF study around the time it claimed success in carrying out thermonuclear weapons tests. While this is not the only fusion technology the country is exploring, the immediate goal of its ICF research appears to be developing a computer simulation program that may be used as a part of a stockpile management system.
North Korea’s ICF capability appears to be in the embryonic stage for now, and there are many challenges when it comes to actually acquiring full ICF capability in which experiments could be run. However, the potential applications of fusion technologies to the country’s weapons program are sufficient enough to warrant greater implementation of sanctions in the academic domain to help impede the North’s potential fusion ambitions.
Fusion Technologies and Their Dual-Use Aspects
A fusion reaction between lightweight elements, such as deuterium, tritium and helium-3, forms different element(s) and releases energy. Fusion reactions usually occur at extremely high temperatures and often under great pressure. Such extreme conditions can be easily met within nuclear explosive devices due to the enormous heat and pressures generated from fission reactions. Advanced nuclear weapons, such as thermonuclear and boosted devices, adopt fusion mechanisms to enhance their yields and the efficiency of fissile material utilization. In the civilian domain, creating conditions that are conducive to fusion reactions requires an immense amount of energy input for heating or compressing fusion fuel.
There are three major branches in the field of nuclear fusion: magnetic confinement fusion (MCF), magnetized target fusion (MTF) and ICF.
- ICF (or direct-drive ICF) aims to achieve fusion ignition by striking a fusion fuel capsule (a few millimeters in size) with high-energy beams or lasers to heat and compress it to an extremely high density within a few nanoseconds.[1] There is a subcategory within ICF, called indirect-drive ICF. In this case, a fuel capsule is located at the center of a hollow chamber. Instead of directly striking the fuel, the laser hits the inner surface of the chamber and then the inner surface emanates X-rays that compress the capsule for fusion ignition.
- MCF utilizes magnetic fields to confine fusion fuel within a donut-shaped device, called a tokamak. Instead of applying high pressure, as in the case of ICF, the MCF approach attempts to confine low-density ionized fusion fuel for as long as possible, in some cases, several seconds, making it unsuitable for weapons applications.
- MTF is an intermediary approach between MCF and ICF with proximity to ICF. The MTF method initially confines ionized fusion fuel with magnetic fields and then squeezes the fuel to induce fusion reactions by amplifying magnetic fields within a short period of time, such as a few microseconds. There are many ways to amplify magnetic fields, such as employing the use of capacitor banks and high-explosive-driven MTFs.
For civilian applications, the fusion community considers the tokamak-based MCF to be the most viable candidate for an energy source. Approximately 35 states worldwide are currently collaborating to realize fusion energy at the world’s largest tokamak, the International Thermonuclear Experimental Reactor (ITER), which is located in France. Meanwhile, ICF and MTF are remote options for being feasible energy sources due to a myriad of technical and economic challenges. For example, it is technically challenging to maintain the uniformity of lasers when attempting to strike a fuel capsule. The costs associated with creating the right conditions for achieving fusion ignition through capacitors or high-energy beams are too significant to offset by the currently achievable level of energy output.
In terms of military applications, fissile and fusion materials are burnt within micro or nanoseconds under high heat and pressure within nuclear explosive devices. In this regard, MCF is far from being applicable for military usage due to its reliance on low-density fusion fuel and requiring a long confinement time.[2] However, ICF poses more significant proliferation risks. Utilizing X-rays in indirect-drive ICF is comparable to imploding the secondary stage of thermonuclear devices. The primary fission stage of a thermonuclear weapon generates X-rays that compress the secondary stage and result in a thermonuclear explosion.
Weapons scientists can benchmark their nuclear weapons simulation codes with the data collected from the nuclear tests conducted in the past. Then, the simulation programs and data that have been newly acquired from ICF experiments can provide scientists with the ability to survey the inner-operations of thermonuclear or boosted weapons beyond the current understanding of design features without requiring further full-scale nuclear tests. As an example of ICF for military applications, the National Ignition Facility (NIF) at the Lawrence Livermore Laboratory conducts indirect-drive ICF experiments and computer simulations to support the US Stockpile Stewardship Program (SSP.)
Continue reading on 38 North.